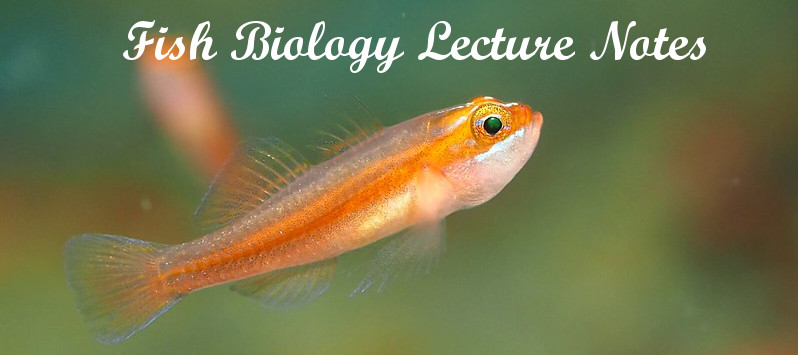
Fish Biology Lecture Notes
This fish biology lecture notes page is complementary to the ichthyology graduate class I taught at the Institute of Environmental and Marine Sciences at Silliman University in Dumaguete, Philippines in 2019/20. It‘s my personal take on the topic, colored by my own intellectual journey to the biology of fishes. Firstly, I grew up as a neurobiologist, and hence the special aspects of fish neurobiology, which set these animals apart from other vertebrates, especially interest me. Second, I live in the Philippines, and I dive and snorkel a lot on tropical coral reefs. Hence, coral reef fishes especially interest me, specifically the gobies.
There are a wealth of topics which I did not cover, simply because of time constrains and because I am not as well read into these topics as into the ones I presented. Skeletal anatomy, trophic ecology, conservation bioloy and metabolism come to mind, all fascinating and important, but left out here. Some topics just drew the short straw – maybe I will cover them in a follow up class someday! Even within the topics I covered, I had to make choices, and these choices are colored by the papers I came along during the last couple of years and my personal interests. For example, there exist a lot of other types of symbiosis involving fishes, but I’m fascinated by cleaner wrasses and I do research on shrimp gobies, so that’s what I covered. And I am sure that there are tons of cool fishes in the Arctic oceans, but that’s not where I dive, so I am talking a lot more about coral reef fishes of the Indo-Pacific.
This is a “best of” of the biology of fishes, a compilation of what impresses me most about fish: The most spectacular evolutionary adaptations, the most unusual life-histories, the most fancy genetic mechanisms unique to fishes, the longest migrations, and the most unusual sensory specializations.
Each section gives a brief outline of the class I taught, with the scientific papers I referenced, and with photographs and videos to complement the text. Some of these photos and videos are my own, or taken from the wealth of educational and scientific materials on the web. You will be able to take in the whole lesson if you work your way through the my lecture notes and the linked or embedded supplementary material. The text I wrote are an outline of the material, and pointers to relevant literature, not an online textbook in itself. A lot of the papers are available online for free, if not you can always contact the authors for a pdf, or find another way to get them. Enjoy!
Evolution – Speciation – Reproduction – Swimming – Symbiosis – Migrations – Senses: Vision – Senses: Audition & Lateral Line – Escape Behavior – Electroreception
Evolution
Fishes are primary aquatic vertebrates, taking up oxygen from the water with gills (with interesting exceptions). All land vertebrates like amphibians, reptiles, birds and mammals are descendants of one specialized lineage of sarcopterygian fishes which transitioned to life on land. The beginning of the evolutionary history of fishes is hence the beginning of vertebrate evolution, and hence the beginning of chordate evolution.
Early Beginnings
All vertebrates are chordates, but primitive chordates are not vertebrates. A chordate is defined by having a chorda, a flexible rod on its dorsal side, and segmented muscles, laterally arranged, plus a dorsal nerve chord. The first chordates were basically swimming worms with a flexible stick along their backs to counteract the lateral muscle forces during swimming. A clever design with a lot of future!
As almost every other phyllum (the highest category in the animal kingdom), the chordates first appeared in the Cambrian, during an event of rapid evolutionary radiation called the “Cambrian explosion”. There is a surprising wealth of fossils from the Cambrian, given that it lies more than half a billion years in the past and that the animals were usually small and soft-bodied. A paper detailing fossils of what are believed to be the very first chordates is this:
There is a lot of art in the interpretation of these fossils!
We are also lucky enough to have one small group of animals survive from these early chordates – the amphioxus, a small marine, filter feeding fish-like animal. The amphioxus’ gene expression in its nervous system is comparable to that of a vertebrate – a clear indication of an evolutionary continuity between these primitive chordates and more derived vertebrates.
This nice figure is from this very insightful review on vertebrate origins:
Next: Skull
Basically, next came the invention of a skull. Again, we are lucky enough to have “living fossils” – the hagfish and the lamprey. The lamprey is a fish-parasite living in freshwater, while the hagfish are deep-sea predators and scavengers.
A very interesting example of hagfish predation, in 91 meters, showing an unusual type of behavior, knotting its own body.
These days we have just a few species, relatively rare (I have never seen one) of these agnathan (jaw-less) fishes, but in Paleozoic times they flourished. Species diversity was high, and larger, heavily armored forms evolved. They all died out by the end of the Devonian, out-competed by their jawed successors…

Jaws (no, not the movie!)
The next step was crucial in the development of all future vertebrates – you probably guessed it, the origin of the jaw. An excellent narrative of this step, and of early fish evolution in general plus of later radations is given in Benton’s book:
Let me make a few points about a few groups – please read Benton’s book for a more complete understanding of these interesting fishes. Wikipedia has a nice overview cladogram detailing the relationships between all these lineages, and when they arose and died out:
An excellent book on the topic is also John Long’s “The Rise of the Fishes”, with many excellent pictures of fossil fishes.
Placoderms – jawed fishes, with heavy bony plates covering their bodies, the bigger ones were the apex predators of their times. The 6 meter long Dunkleosteus was akin to the Great White Shark of Devonian times. Possibly they were out-competed by sharks and hence died out. The placoderms were close to the first jawed fishes.
Klug, C., Frey, L., Pohle, A., de Baets, K., & Korn, D. (2017). Palaeozoic evolution of animal mouthparts. Bulletin of Geosciences, 92(4), 511–524.
While the placodermes swam in the oceans of the Devonian and early Carboniferous, a very, very long time ago, they were already far from “primitive fishes”. Besides some of them probably being fearsome predators, the males had developed claspers for internal fertilization:
Trinajstic, K., Boisvert, C., Long, J., & Maksimenko, A. (2014). Pelvic and reproductive structures in placoderms ( stem gnathostomes ).
And the females carried the developing embryos inside their bodies, possibly the first examples of internal fertilization and development in vertebrates:
Long, J. A., Trinajstic, K., & Johanson, Z. (2009). Devonian arthrodire embryos and the origin of internal fertilization in vertebrates. Nature, 457(7233), 1124–1127.
Acanthodii – a group which flourished in the Paleozoic, sometimes called the “spiny sharks”, due to the fact that they had features of bony fishes and sharks. A successful group f mid-sized to smaller fishes in the Paleozoic, which however died out by the late Carboniferous.
Sarcopterygii – the lobe fined fishes are close to the first land animals (tetrapods). Presently, only a few species of “living fossils” remain on deep reefs, while in the Paleozoic they were very species rich, and dominated many marine and freshwater ecosystems, often with large-bodied species. Note the stem of the pectoral and pelvic fins of the fish in the video below.
A bit of a longer clip, featuring some super amazing tech dives in South Africa where the divers encountered a Coelachant. The Coelachant footage starts at 15:00, but the whole video is interesting to watch, some nice views of the mesophotic zone.
Sharks, Rays and Chimeras – we all know the Great White Shark and the Tiger Shark and several other large, carnivorous, torpedo-shaped sharks. There are some bottom-dwelling and some deep sea species which look and feed very different, but in many shallow-water ecosystems these days few shark species live, and these are often large and mostly near the top of the food chain. This was not always the case, in the famous fish assembly preserved at Bear Gulch from the lower Craboniferous of Montana, sharks and chimeras dominate the fauna, with more species than bony fishes and with many morphologically very diverse forms.
Rays were a late branching lineage evolved from sharks.

Bony Fishes, esp. Teleosts – Almost every animal we know these days as a fish is a teleost bony fish. Gars, bowfins, birches and sturgeons are some of the few bony fishes outside of the teleosts. The teleosts are set apart from other bony fishes by more movable, protrusible jaws. Many teleost lineages also have evolved secondary, pharyngeal jaws deeper inside their jaws. Teleosts underwent a massive radiation, and include fishes as diverse as a tuna and a seahorse. The exact relationships within the teleosts are still under discussion.
Proscinetes from the Jurassic, a Pycodontiform bony fish, a group distinct from today’s from the teleosts. Note the solid spherical teeth, the fish was most likely a shell-crusher.
My own research last year led me to look at the evolution of large pelagic filter feeders, like baleen whales or basking & whale sharks – most, but not all of them were fishes throughout evolutionary history. The laminid sharks are highly over-represented in this niche.
Speciation
What we discussed above is macroevolution, the half-billion-year journey from primitive stem chordates to advanced teleost fishes. When we look at evolution at a smaller time-scale, the question becomes how new species evolve – a process called speciation.
The late great Ernst Mayr contributed a lot to the modern understanding of speciation. Basically, a sub-population of a species needs to be isolated from the rest so that new adaptive innovations can take hold, and that genetic drift can separate the populations out. When the sub-populations are separated by spatial distance, that is called para- or peri-patric speciation. But can new species also evolve without spatial separation, when behavior creates a barrier? This is called syn-patric speciation.
I want to point to a few excellent studies which investigated speciation in fishes:
Speciation by a change in the electric mating call in African elephant fishes: A change in the signal used to attract females separates two populations:
Arnegard, M. E., McIntyre, P. B., Harmon, L. J., Zelditch, M. L., Crampton, W. G. R., Davis, J. K., … Hopkins, C. D. (2010). Sexual Signal Evolution Outpaces Ecological Divergence during Electric Fish Species Radiation. The American Naturalist, 176(3), 335–356.
In gobies, subtle differences in the ambient light in the fish’s environment lead to different eye pigments and drive species apart:
Larmuseau, M. H. D., Huyse, T., Vancampenhout, K., Van Houdt, J. K. J., & Volckaert, F. A. M. (2010). High molecular diversity in the rhodopsin gene in closely related goby fishes: A role for visual pigments in adaptive speciation? Molecular Phylogenetics and Evolution, 55(2), 689–698.
In African cichlid fishes, speciation comes about when the light conditions are distinct – when fishes live at different depth levels. These differences in vision also lead to changes in the nupial coloration of males. A fascinating interaction between environmental (light) conditions, genes, retinal pigments, body coloration and mating behavior which drives species apart:
Reproduction
Okuda, N., & Yanagisawa, Y. (1996). Filial cannibalism by mouthbrooding males of the cardinal fish , Apogon doederleini , in relation to their physical condition, (Mrowka 1987).
This nice video by GwynnbrookFarm shows the behavior:
This is a good general description of the behavior:
Buckley, J., Maunder, R. J., Foey, A., Pearce, J., Val, A. L., & Sloman, K. A. (2010). Biparental mucus feeding : a unique example of parental care in an Amazonian cichlid, 3787–3795.
This study analyzes the mucus: it’s only produced when the parents take care of fry. It also contains valuable information about the precise composition (protein & mineral rich) of the mucus:
Chong, K., Sock, T., Foo, J., Toong, L., & Chong, A. (2005). Characterisation of proteins in epidermal mucus of discus fish ( Symphysodon spp .) during parental phase.
Deep-sea anglerfishes have evolved what is probably the most curious example of reproductive specialization in fishes. The females are mid-sized fishes, often about the size of a fist; in contrast the males are among the tiniest vertebrates, with lengths of only 6 mm in some species! Once a male finds a female, in several species, attaches to her and reduces most bodily systems other than his gonads. What a life
This paper gives a good overview over the evolution of this male parasitism (it evolved multiple times), and about the differing degrees of male attachment/organ reduction:
What should be mentioned in the context of fish reproduction is the role reversal seen in seahorses and pipefishes (Syngnathidae): Here, the males take care of the fertilized eggs, by either keeping them in a pouch or by attaching them to their ventral sides. This leads to a role reversal (at least in some cases): the females, who now invest less biological energy into reproductions need to vow the males. Things are not straight forward, s usual, as not all syngnathids reverse sex roles, this happens more often when a female mates with more than one male:
Sharks: Smaller, evolutionarily more basal sharks like horn sharks lay eggs. These are typically larger eggs, with tough outer skins. Hence these species already tend into the K-direction (smaller offspring numbers, more investment per offspring) along the K-R continuum.
More advanced sharks like the sand tiger sharks show something called “intra uterine cannibalism”, where the larger embryos feed on their less developed brothers and sisters in the female shark’s womb. These are some sand tiger sharks (as they are called in the US, ragged tooth sharks in South Africa and Grey Nurse sharks in Australia, Carcharias taurus scientifically – this is why we have scientific names!):
Even more highly evolved sharks, like the great hammerhead shark have again evolved evolutionary strategies converently similar (similar in function but not in evolutionary origin) to mammals. The female hammerhead shark feeds its offspring in the womb with a placenta-like structure. This paper goes into detail on the hammerhead placenta:
Finally, my own contribution to the field of fish reproduction. Why are There No Eusocial Fishes? Eusociality, the form of reproductive specialization seen in ants, bees, termites and many other species including a mammal (the naked mole rat) does NOT occur in fishes, while so many other unusual reproductive strategies occur in fishes. Why? I argue that it’s due to the boundary conditions in the ocean, mainly due to much stronger larval dispersal.
Stiefel, K. M. (2013). Why are there no eusocial fishes?. Biological Theory, 7(3), 204-210.
Conclusion: suckling & placentae like in mammals, and unusual tricks like mouth brooding and parasitic males, fishes have it all. And we haven’t even covered the sex changes seen in many wrasses & some damselfishes, the “sneaker males” in some wrasses and groupers.
Swimming
Swimming is hard, and I am not talking about the difficulty of propelling yourself forward when you find yourself in the ocean in a spot where it’s too deep to stand; the difficulty is in understanding fish locomotion, since fluid dynamics is one of the hardest problems left in physics. Once turbulences start happening, it’s very hard to predict how body shapes will influence drag. One key concept in fluid dynamics is the Reynolds Number, explained in this video:
There are a variety of ways which fishes use to push themselves through the water. This paper explains them well:
This is the main figure of the paper, which illustrates the different swimming modes of fishes:
Basically, the basal swimming mode is to undulate all laterally with all of your fish body. The more effective and faster ways of swimming then move less and less of the body, and more and more only transfer the energy of teh muscles to the caudal fin. Then, a number of locomotory modes exist which are not optimized for speed, but for maneuverability, these undulate or oscillate use either the dorsal, anal or pectoral fins. It’s greatly instructive to keep this diagram in mind when going for a snorkel on a coral reef and observing different fish.
Also worth reading is:
Blake, R. W. (2004). Fish functional design and swimming performance. Journal of fish biology, 65(5), 1193-1222.
Very interesting are shark skins. Tiny “riblet structures” cover the skins of sharks, which seem to serve the purpose of “managing” the eddies which occur along side larger animals at higher speeds. The ribblets vary between species and between spots on the body within one shark species:
B. Dean and B. Bhushan Shark-skin surfaces for fluid-drag reduction in turbulent flow : Phil. Trans. R. Soc. A (2010) 368, 4775–4806
Conclusion: The locomotion of fishes is almost as diverse as is fish phyllogeny itself. And we didn’t even touch on non-swimming types of fish locomotion like terrestrial locomotion by mudskippers, and underwater walking of some frogfishes and stone fishes, like this one:
Symbiosis
Symbiosis is simply the interaction between animals of 2 species on a regular basis – there can be parasitism (advantage/disdvantage), commensalism (advantage/neutral) and mutualism (advantages for members of both species involved). Sometimes the lines between these can be blurry!
In my lecture, I covered two kinds of mutualistic (?) symbiosis which fish engage in: cleaning behavior and the shrimp-goby symbiosis.
Cleaning
An interesting symbiosis seen in coral reef fishes is cleaning. Reef fishes are subject to a heavy load of parasites, mostly copepod and isopod crustaceans. Cleaner wrasses inspect larger fishes and remove these parasites. The process looks like this:
Curiously, wrasses are not always honest cleaners. They often/sometimes cheat and take fish mucous instead of parasites. A tasty meal for them, but a harmful episode for the client in need of cleaning. When they cheat, they sometimes use predatory fish as “cover” to avoid punishment by the cheated clients; and they recognize clients they have interacted with:
Oates, J., Manica, A., & Bshary, R. (2010). The shadow of the future affects cooperation in a cleaner fish. Current Biology, 20(11), 472–473.
Zealand, N. (1995). Cleaning Symbiosis as an Evolutionary Game : To Cheat or not to Cheat?
Wismer, S., Grutter, A., & Bshary, R. (2016). Generalized rule application in bluestreak cleaner wrasse ( Labroides dimidiatus ): using predator species as social tools to reduce punishment. Animal Cognition.
The bicolor cleaner wrasses, which do not maintain “cleaner stations” at set spatial locations are somewhat mroe inclined to cheat:
Mills, S. C., & Côté, I. M. (2010). Crime and punishment in a roaming cleanerfish. Proceedings of the Royal Society B: Biological Sciences, 277(1700), 3617–3622.
It’s all game theory! What decisions do you have to make to optimize your success in the long run, with limited knowledge, while you are facing an adversary who is faced with the same dilemma?
This need to read the state of minds of other fishes has made the wrasses develop advanced cognitive skills. They can pass the “mirror test”:
Id, M. K., Id, T. H., Takeyama, T., Awata, S., Tanaka, H., Asai, J., & Jordan, A. L. (2019). If a fish can pass the mark test , what are the implications for consciousness and self- awareness testing in animals ?
Waal, F. B. M. De. (2019). Fish, mirrors, and a gradualist perspective on self-awareness.
This advanced cognitive capacity is likely due to an improved structural complexity of some forebrain nuclei, not due to generally larger brains:
Chojnacka, D., Isler, K., Barski, J. J., & Bshary, R. (2020). Relative Brain and Brain Part Sizes Provide Only Limited Evidence that Machiavellian Behaviour in Cleaner Wrasse Is Cognitively Demanding, 1–14.
Shrimp-gobies
There are over 100 species of gobies which share burrows with alpheid shrimp. The shrimp digs a burrow, the goby and the shrimp use this burrow to hide from predators, and the goby warns the shrimp of approaching predators via a tactile communication system: the shrimp wiggles its tail, or rapidly escapes into the borrow, when a predator comes close, or very cloase.
This is an excellent review of the topic:
Karplus, Ilan, and Andrew Richard Thompson. “The partnership between gobiid fishes and burrowing alpheid shrimps.” The biology of gobies. Science, Boca Raton, FL (2011): 559-608
Since the shrimp-goby symbiosis is one of my active topics of research, and I have set up the Shrimp Goby Page about the interesting symbiosis, featuring a more in-depth write up and many images and videos.
This symbiosis is so successful, and the starting position (shrimp which likes to dig, goby which likes to hide in burrows) is so conductive to its development that the symbiosis evolved twice in the Indo-Pacific:
Thacker, C. E., Thompson, A. R., & Roje, D. M. (2011). Molecular Phylogenetics and Evolution Phylogeny and evolution of Indo-Pacific shrimp-associated gobies ( Gobiiformes : Gobiidae ). Molecular Phylogenetics and Evolution, 59(1), 168–176.
An interesting example of an evolutionary intermediate is found in the Caribbean, where a species of goby is only facultatively associated with burrowing shrimps:
Kramer, A., Tassell, J. L. Van, & Patzner, R. A. (2009). A comparative study of two goby shrimp associations in the Caribbean Sea, 137–141.
A bit of shrimp goby footage from the northern Philippines.
Photos
Below is a collection of photographs I have taken during the last couple of years of fishes involved in mutualist symbiosis, mainly the anemonefishes, shrimp gobies and cleaner wrasses.
Migrations
Fish don’t spend all of their lifes in the same spot, and instead sometimes migrate amazingly long distances. This can be for feeding, mating, or escape from predation.
A female great white shark was tagged with a ta which sent position and depth information to a satellite (once the shark breached), and she was found to swim from Western Australia to South Africa, and back, in a span of about three months. This was especially surprising since genetic data had indicated that female GWS do not migrate long distances. And, she also dived up to 1000 m deep during that migration!
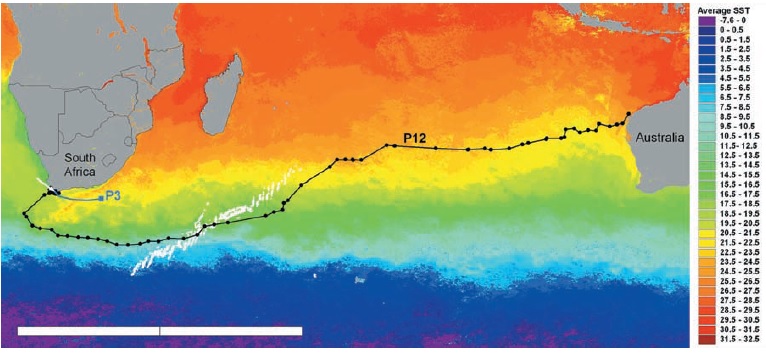
Manta rays migrate as well, over significant parts of the Red Sea, as seen in this study: The migrations are not as extensive as with GWS, but nevertheless substantial, and their dives also lead them to ~ 700 meters. Note the “gliding” phases during their migrations, this connects to teh chapter about swimming physics.
Some manta rays, from Yap.
Let’s also look at the different ways fish migrate from and to freshwater and the ocean (nice figures in the link):
– amphidromous: larvae in the ocean, the rest of the life in rivers or lakes: many gobies:
– anandromous: mating in freshwater, growth in the ocean, return to freshwater before mating, as in salmon.
– catadromous: life in freshwater, return to the ocean for mating, as in many eels.
There are connections between these life-styles, and they are not what they seem at a first glance. Catadromous migrations might be a more extreme case of amphidromous migrations:
Version, D. (2013). There and back again: migration in freshwater fishes. Canadian Journal of Zoology
An interesting example of anandromous migrations is described in this paper:
Berdahl, A., Westley, P. A. H., & Quinn, T. P. (2017). Social interactions shape the timing of spawning migrations in an anadromous fi sh. Animal Behaviour, 126, 221–229.
Salmon seem to start moving up a river based on a voting system among the fish. Only when a significant number of fish initiate a migration do others follow. The paper is a clever combination of observations and computer simulations of migration statistics. Another point I’d like to make: I first came across this work when reading an article in the quality popular science magazine Hakai (I’ve also written for them in the past). Science journalism on this level can be a good way to find interesting new work in a huge sea of constantly appearing new papers.
Vision
Fish neurobiology is a rich field. There are two reasons for this: Some excellent science was done with scientists having fish biology in mind. Other work was done with fish used as “model systems”: A lot of the work done on teh zebrafish, for instance, was not primarily motivated by wanting to know about fish, but by wanting to know about developmental biology or neural wiring, in general. As fish biologists, we can enjoy this knowledge either way.
As in all other vertebrates, the fish visual system receives light onto the photoreceptor cells of the retina. The retina then sends the visual signal onto the thalamus (and from there to the forebrain), and onto the superior colliculus. This is a super-super short version of visual processing, of course. If you’d like to learn more about the vertebrate visual system in general, Principles of Neural Science is a good choice. This is a good intro more specific to bony fishes:
Bakken, T. E., & Stevens, C. F. (2012). Visual System Scaling in Teleost Fish, 153, 142–153.
An interesting study detailing the very fine-grained way of processing visual information in the zebrafish superior colliculus is this:
To stick with the theme of the course, which is to emphasize the biological adapatations which are special to fishes, I would like to point you to this paper:
Warrant, E. J., & Locket, N. A. (2004). Vision in the deep sea, 671–712.
Deep sea fishes are living in regions of the ocean where light is extremely sparse. As a consequence, they need to do their very best to harvest every single photon which comes their way. Multiple stacked layers of photo-receptor cells, or vertically enlarged photoreceptor cells are one solutions. Large eyes and specialized lenses are another.
Audition and Lateral Line Senses
Fish can hear, like land mammals, however they need to perceive sound waves in a very different environment, in water, which is many times denser than air. They also have a sense which detects proximal water movements, the lateral line sense. These senses share common central nervous system structures.
The lateral line is composed of superficial, or slightly depressed sensory cells (neuromasts) which sense water movement. The IIX brain nerve (“octavolateralis”) transmits all auditory and most lateral line information to the central nervous system. A great resource for the understanding of fish hearing is this book:
Fay, R. R. , A.N. Popper (Ed.). (1998). Comparative hearing: fish and amphibians (Vol. 11). Springer Science & Business Media.
You can read it on Google Books. Chapter 8 in this book gives an excellent overview of the lateral line system, including the different physics of water (mass) movement which it picks up, in contrast to the sound waves, which propagate vibrational energy, but not water molecules.
Chapter 3 in Fay & Popper’s book gives a nice overview of the fish inner ear, with its liquid-filled vesicles and resonator-stonelets, the otholits.
Interesting evolutionary specializations exist in the bony fish when it comes to peripheral auditory structures. The swim bladder is a great auditory resonator. A large group of teleosts are included in the Ostariophysi,and they share a special set of bones, the Weberian organ, which connects the swimbladder to the inner ear.
The Weberian organ is derived from anterior vertebrae, these papers outlines its development and function:
This improved hearing gives the Ostariophysi a huge advantage, and they are a very successful group. Especially the catfish dominate many freshwater habitats. Jimi Hendrix already knew about the auditory capabilities of catfish, and wished he was one:
Semi-funny jokes aside, as in so many other instances, we see that behavior & sensory performance are drivers of evolution.
It seems that whenever you want to see some curious, advanced adaptations in fish, you should look to the Cichlids. Some Cichlids – which are not a part of the Ostariophysi and hence lack Weber’s organ – have extended swimbladder protrusions which connect to the inner ear. This is an alternative solution to the Weberian organ. This paper shows these swimbladder modifications in amazing detail:
Schulz-mirbach, T., Metscher, B., & Ladich, F. (2012). Relationship between Swim Bladder Morphology and Hearing Abilities – A Case Study on Asian and African Cichlids, 7(8).
The swimbladder (green) and the inner ear (purple, red), in close contact in the Cichlid Etroplus maculatus. Figure from the paper cited above.
Escape Behavior
Herbert Spencer coined the term “survival of the fittest”, and its aptness is well demonstrated in the escape system of bony fishes. If you as a fish don’t have a good escape system, you ain’t fit, and you won’t survive! The escape system has been investigated over the last decades by a number of labs, with a variety of methods. The result is an interesting body of neurobiological and neuroethological (the study of behavior and its neural causes) work.
Escape reflexes are diverse in among fish phyllogeny: Lionfish and rockfish turn, to aim their venomous spines towards a threat. Eels retreat into a crevice; There is also the “typical escape reflex”, as seen in many free-swimming fishes, such as goldfish or zebrafish, two species in which this behavior is extensively researched. This works like this: Phase one, starting at 5 ms, is the stereotypical C-start reflex initiates, where the fish bends rapidly in one direction. This is followed by a second phase where the fish bends back into the opposite direction, and swims away into a (seemingly) random direction.
This is one of the classic studies in the field, with a very thorough description of the behavior:
Eaton, R. C., Lavender, W. A., & Wieland, C. M. (1981). Identification of Mauthern-initiated response patterns in goldfish: evidence for simultaneous cinématography and electrophysiology. Journal of Comparative Physiology, 144, 521–531.
This behavior is neuronally implemented by the Mauthner cell system; The hindbrain Mauthner cell has been called a “command neuron”, in the sense that it integrates sensory information (auditory, lateral line and visual) and gives a fast command to the motor system (spinal chord).
However, there seems to be some redundancy, and a weakened escape reflex persists without Mauthner neurons:
Nissanov, J., Eaton, R. C., & DiDomenico, R. (1990). The motor output of the Mauthner cell, a reticulospinal command neuron. Brain Research, 517(1–2), 88–98.
Eaton, R. C., Lee, R. K. K., & Foreman, M. B. (2001). The Mauthner cell and other identified neurons of the brainstem escape network of fish, Progress in Neurobiology 63, 467–485.
A number of interesting studies elucidating different aspects of the Mauthner cell system:
Social status influences the escape reflex:
Miller, X. T. H., Clements, K., Ahn, X. S., Park, X. C., Ji, X. E. H., & Issa, X. F. A. (2017). Social Status – Dependent Shift in Neural Circuit Activation Affects Decision Making, 37(8), 2137–2148.
Additional interneurons contributing:
Lacoste, A. M. B., Schoppik, D., Robson, D. N., Haesemeyer, M., Portugues, R., Li, J. M., … Schier, A. F. (2015). A Convergent and essential interneuron pathway for mauthner-cell-mediated escapes. Current Biology, 25(11), 1526–1534.
Damselfish escape reflex variability:
Jornod, M., & Roche, D. G. (2015). Inter- vs intra-individual variation and temporal repeatability of escape responses in the coral reef fish Amblyglyphidodon curacao, 20, 1395–1399.
Finally, to indulge in more neuroethnological diversity, let’s look at slow-motion footage I managed to capture of sand divers (Trichonotidae), very unusual fishes with a specialized escape behavior which constitutes in diving under the sand:
Electroreception
Some fish can sense electric fields, some can produce them, and some can both. This gives them a sense which no other animals on this planet have. In the last part of the course we’ll look at these “weak electric fishes”.
A number of fish orders can sense electric fields; this is a very useful capability if you are underwater, where electric fields propagate much better than in air; air is a very poor conductor of electricity.
Sharks and rays sense electricity with their ampullae of Lorenzini, which are small, gel-filled canals mostly located on the head of the shark or ray. You can see them here on the underside of this sandtiger shark, the dark spots:
Interestingly, Stefano Lorenzini of Florence already discovered these ampullae in 1678, at a time when many of the advanced anatomical techniques like microscopy and stains were not available yet; however a keen observer still had the chance to make discoveries.
On a side note, Lorenzini and his brother, a mathematician, were imprisoned by Grand Duke Cosimo III de’ Medici, who imprisoned him along with his brother Lorenzo Lorenzini, a famous mathematician. An early interdisciplinary meeting, in jail! Also, an early episode of the authorities appreciating scientists! /irony off
Electrosensitive organs can be divided into tuberous and ampullary organs; the ampullary organs are more sensitive, but are stronger low pass-filters. The weak-electric fishes (see below) need to sense high-frequency signals, and hence have tuberous organs as well.
This paper about the ampullae of Lorenzini in a freshwater ray is illustrative for two reasons: It contains some really nice electron microraphs, and since these rays live in freshwater, with lower electrical conductivity. Hence, by looking at the altered shape (shorter) of the ampullae, we can see a structure-function relationship.
Several lineages of catfish can also sense electric fields; as well as several smaller lineages.
Also, several groups of fishes can generate electric fields, such as the “numb rays”, and the stargazers. This is done by stacking the fields of modified muscles, or, in rare exceptions, modified nerve fibers. Any muscle contraction generates an electric field, and a lot of muscles stacked generate a stronger field; if you take away the actual contraction mechanism, you have a dediceated electric pulse generator.
Things get really interesting when fish can do both: generate and sense electric fields. This evolved twice, in South American knife fishes (Gymnotiformes), and in African elephant fishes (Mormyridae). These are the “weak electric fishes” – their electric fields are weak, not meant to stun, but to detect. In many of the rivers and lakes which they inhabit, they are dominant in terms of the number of species. As so often, behavior and perception drive evolution.
This phyllogenetic reasoning of fish electricity briefly outlined above is well laid out in this paper:
Alves-Gomes, J. A. (2001). The evolution of electroreception and bioelectrogenesis in teleost fish: A phylogenetic perspective. Journal of Fish Biology, 58(6), 1489–1511.
These “weak electric fishes” have an active electric sense, they are capable of sensing the distortion caused by the environment of their self-generated electric fields. These fields can range in frequency > 1000 Hz, and such high frequencies allow very precise determination of the happenings in their environment, both in time and space. This simulation paper illustrates that:
Nelson, M. E., & MacIver, M. A. (1999). Prey capture in the weakly electric fish Apteronotus albifrons: sensory acquisition strategies and electrosensory consequences. Journal of Experimental Biology, 202(Pt 10), 1195–1203.
How are such fast oscillations generated? In the pacemaker nucleus, one of the most precise structures known to neuroscience. An ace paper from my old lab at Salk, showing how gap junctions play a big role in the precision of this nucleus:
Moortgat, K. T., Bullock, T. H., & Sejnowski, T. J. (2000). Precision of the pacemaker nucleus in a weakly electric fish: Network versus cellular influences. Journal Of Neurophysiology, 83(2), 971–983.
The weak electric fishes have huge brains in relation to their body sizes, since the distortion of these very fast fluctuating field. It’s a modified cerebellum which has evolutionarily grown so much in these fishes. The structure where the signal initially arrives is the electrosensory lateral line lobe.
A well-studies behavior in weak electric fish is the Jamming Avoidance Response. When two electric fish use the same frequency in their electric sensing, that would lead to signal jamming. To avoid that, a reflex has developed which causes the frequencies to move apart. Basically, the fish brains compare amplitude/phase envelopes. How that works in South American and African weak electric fish is described here:
Kawasaki, M. (1996). Comparative analysis of the jamming avoidance response in African and South American wave-type electric fishes. Biological Bulletin, 191(1), 103–108. https://doi.org/10.2307/1543070
This is my favorite weak electric fish paper:
Just as there is am attentional spotlight in the visual system of mammals, there seems to be an attentional spotlight in the electrosensory lateral line lobe of gymnotids: This is another indication how highly developed these knife fishes, one of the apexes of vertebrate evolution, are:
Berman, N., & Maler, L. (1999). Neural architecture of the electrosensory lateral line lobe: adaptations for coincidence detection, a sensory searchlight and frequency-dependent adaptive filtering. The Journal of Experimental Biology, 202(# (Pt 10)), 1243–1253.
Finally, let’s look at the swimming style of a knife fish, as already discussed in the section about swimming. The anal fin oscillates, but the body of the fish remains stiff. This is the case since the body of the fish serves as a 3d-sensor for electric fields, and could not function well at that if it changed shape much: